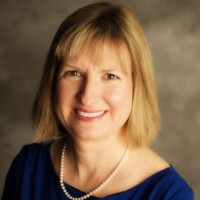
Biography
Margaret Frey is the Vincent V.C. Woo Professor in the department of Fiber Science & Apparel Design at Cornell University. She is a Faculty Fellow for Atkinson Center for a Sustainable Future and the Barbara McClintock Residence Hall at Cornell University.
Professor Frey earned a BS in Chemical Engineering and an MS in Fiber Science from Cornell University. She earned her PhD in Fiber & Polymer Science from NC State University.
My research in the area of micro and nanofibers has focused on potential uses for high specific surface area materials with tailored surface chemistry. In applications ranging from air and water filtration to lab-on-chip micro chemical analysis systems, I have demonstrated that functional surfaces for capture and isolation of specific compounds can be effectively created using nanofibers. Nanofibers have the unique advantages of high surface to volume ratios, easy handling and compatibility with a wide range of substrates including textiles, plastics, papers and metals. I have made significant contributions in developing nanofibers as functional surfaces and structures for microfluidic systems. Nanofibers invented in my laboratory can perform important functions including sample purification, analyte concentration and reagent mixing in microfluidic channels. These nanofibers perform better than the generally used structures produced via expensive and slow gold lithography processes which must be performed in a clean room.
Compared to conventional fibers in conventional textile applications, nanofibers have inherent draw backs, including slow production rate, high cost, and poor abrasion resistance. However, in comparison to other high surface/low volume materials typically produced by lithographic methods, nanofibers have huge advantages. These advantages include simplicity of production without requiring a clean room, great material flexibility to create a wide range of surface chemistries and material patterns, and comparatively low cost. In collaboration with colleagues from the Colleges of Agriculture and Life Sciences and Engineering, I have developed unique fiber solutions for agricultural chemical delivery, microfluidic diagnostic devices and lateral flow assay systems. Based on the needs of specific systems,we have been able to develop a broad array of nanofiber functionalities, including hydrophilic and hydrophobic surfaces, positively and negatively charged surfaces, chemically active surfaces, and conductive and piezo electric fibers. All of these functionalities have beendemonstrated in model devices.
In my research, micro and nanofibers are produced primarily by electrospinning with a focus on using the process variables to drive final morphology of individual fibers and nonwoven membranes. The strong elongational flow field, electrical gradient and thermodynamics of solvent evaporation and polymer phase separation all contribute to production of fibers with fine diameters, high concentration of active components at the fiber surface and membrane structures ranging from random to well aligned. In a one step process utilizing phase separation, functional materials including small molecules, non-fiber forming polymers and proteins are added to nanofibers. By co-dissolution or suspension of the active material with a fiber forming polymer, nanofibers combining the desired surface chemistry with good mechanical properties and uniform morphology are produced rapidly and reproducibly . Additionally, post spinning methods, including layer-by-layer deposition, covalent bonding and polymerization of a second material via gamma grafting or vapor phase deposition, have been utilized to add additional active properties. Nanofiber membranes have been further incorporated into larger structures by directly spinning into microfluidic channels or cutting shapes from larger membranes for use in microfluidic channels, lateral flow assay devices, filtration systems or agricultural trials.
FSAD 1111: Success in Fiber Science & Apparel Design
FSAD 1350: Fabrics, Fibers and Finishes
FSAD 1360: Fiber Laboratory
FSAD 2370: Structural Fabric Design
FSAD 6660: Fiber Formation Theory and Practice
IGERT Module: Sustainable Industry Practices
IGERT Module: Nanomaterials for Biosensors
Senior Associate Dean, Undergraduate Affairs
Interim Chair, Fiber Science & Apparel Design
Faculty Fellow, Cornell Institute for Fashion and Fiber Innovation
Faculty Fellow, Atkinson Center for a Sustainable Future
1985, B.S. , Chemical Engineering, Cornell University
1989, M.S., Fiber Science, Cornell University
1995, Ph.D., Fiber and Polymer Science, North Carolina State University